What is Glycogen, What is its Structure, Function and Metabolism?
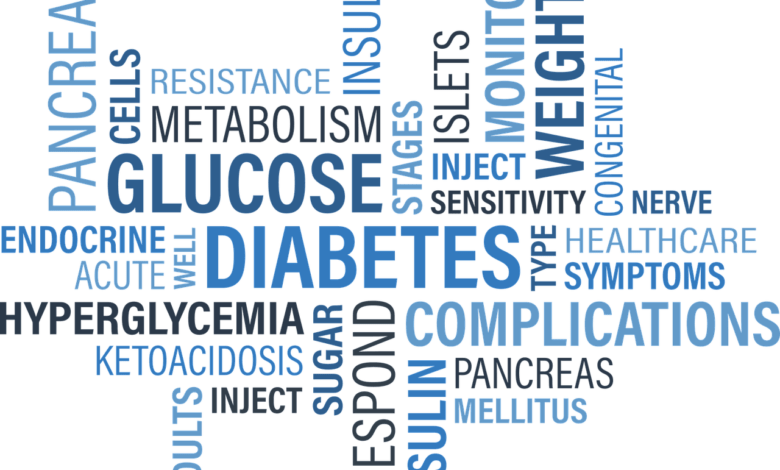
Glycogen is a large, branched polysaccharide that is the main storage form of glucose in animals and humans. It is a complex polymer made up of multiple chains of glucose molecules and is found in all cell types except erythrocytes. Its main organs are the liver and skeletal muscles. Glycogen is also an important form of glucose storage in fungi and bacteria. When the body requires energy, glycogen breaks down into glucose, then enters the glycolytic or pentose phosphate pathway or is released into the bloodstream.
Structure of Glycogen
Glycogen is a branched polymer composed of glucose. Glucose residues are linearly linked by α-1,4 glycosidic linkages and approximately one chain of glucose residues every ten residues branches through α-1,6 glycosidic linkages. A-glycosidic bonds result in a helical polymer structure. Glycogen is hydrated with three to four parts of water and is present in the cytoplasm in the form of granules 10-40 nm in diameter. The enzyme called glycogen, which is involved in glycogen synthesis, is at the center of each glycogen granule. Glycogen is a starch analog that is the main form of glucose storage in most plants, but starch has fewer branches and is less compact than glycogen.
The Function of Glycogen in Living Things
Glycogen is found mainly in muscle and liver cells in animals and humans. Glycogen is synthesized from glucose when blood sugar levels are high and serve as a ready source of glucose for tissues in the body when blood sugar levels drop. Glycogen is not as reduced as fatty acids and therefore not rich in energy. Glycogen is an important fuel reserve for several reasons. The role of glycogen in maintaining blood glucose levels is particularly important because glucose is the only fuel used by the brain other than prolonged fasting. Glucose from glycogen is easily mobilized, so it’s a good source of energy for sudden, strenuous activities. Released glucose can provide energy in the absence of oxygen and thus provide energy for anaerobic (oxygen free) activity.
Glycogen in Liver Cells
The glycogen concentration is higher in the liver than in the muscle, it constitutes 6-10% of the liver by weight. When food is taken into the body, blood sugar levels rise and insulin released from the pancreas promotes the uptake of glucose into liver cells. Insulin also activates enzymes involved in glycogen synthesis, such as glycogen synthase. While glucose and insulin levels are high enough, glycogen chains are lengthened by the addition of glucose molecules, a process called gluconeogenesis. As glucose and insulin levels decrease, glycogen synthesis stops. When blood glucose sugar levels drop below a certain level, glucagon released from the pancreas signals the liver cells to break down glycogen. Glycogen is broken down into glucose-1-phosphate through glycogenolysis, which is converted into glucose and released into the circulation. The controlled breakdown of glycogen and the release of glucose increases the amount of glucose available between meals. This is why glycogen acts as a buffer to maintain blood glucose levels. In addition to glucagon, cortisol, epinephrine, and norepinephrine also stimulate glycogen breakdown.
Glycogen in Muscle Cells
Unlike liver cells, glycogen constitutes 1-2% of the muscle mass by weight. Given a larger muscle mass in the body, the total amount of glycogen stored in muscle is greater than that stored in the liver. Muscle is also different from the liver, in that the synthesis and degradation of glycogen are regulated in the liver to maintain blood sugar levels necessary to meet all the needs of the organism. In contrast, muscle glycogen only supplies glucose to the muscle cell itself. Produced from the breakdown of glycogen in muscle fibers, glucose-1-phosphate is converted into the glucose-6-phosphate molecule and provides energy to muscles when exercising or in response to stress, as in a fight or flight response.
Glycogen in Other Tissues
Glycogen is found in smaller amounts in other tissues, including red blood cells, white blood cells, kidney cells, and some glial cells, in addition to the liver and muscles. Glycogen is also used to store glucose in the womb (uterus) to meet the energy needs of the embryo.
Glycogen in Fungi and Bacteria
Microorganisms have energy storage mechanisms to cope in case of limited environmental resources and glycogen is the main form of energy storage. While nutrient restriction (low levels of carbon, phosphorus, nitrogen or sulfur) can stimulate glycogen formation in yeast, bacteria synthesize glycogen in response to carbon energy sources that are readily available by limiting other nutrients. Bacterial growth and yeast sporulation (spore formation process) have also been associated with glycogen accumulation.
Glycogen Metabolism
Glycogen homeostasis is a highly regulated process that allows the body to store or release glucose depending on its energy needs. The regulation of glycogen metabolism is mediated by hormonal activities, especially insulin, which lowers blood sugar to normal, glucagon and epinephrine, which help to raise blood sugar. Key steps in glucose metabolism are glycogenesis (glycogen synthesis) and glycogenolysis or glycogen breakdown.
Glycogenesis: Glycogen synthesis requires an activated form of glucose, uridine diphosphate glucose (UDP-glucose), formed by the reaction of uridine triphosphate (UTP) and glucose 1-phosphate. Glycogen synthesis includes five reactions:
Conversion of 1-glucose 6-phosphate to glucose 1-phosphate
Synthesis of UDP-glucose from 2-Glucose 1-phosphate and UTP
(UDP-glucose is attached to the non-reducing end of glycogen molecules.)
3- Automatic catalyzed synthesis of a glucose oligomer on glycogen
4- Linear extension of glucose oligomer catalyzed by glycogen synthase
5- Formation of branches catalyzed by glycogen branching enzyme
Repeating the last two reactions produces large, widely branched glycogen polymers.
Glycogenolysis: Glycogen breakdown consists of three stages:
1-Release of glucose 1-phosphate from glycogen
2-Remodeling of glycogen substrate to allow further degradation
3- Conversion of glucose 1-phosphate into glucose 6-phosphate for further metabolism
Glucose 6-phosphate derived from the breakdown of glycogen can be used in three ways:
1-It is the first substrate for glycolysis.
2- It can be processed via pentose phosphate to give NADPH and ribose derivatives
3-It can be converted into free glucose to be released into the bloodstream. This conversion occurs mainly in the liver and to a lesser extent in the intestines and kidneys.
Exercise and Glycogen Deficiency
In endurance exercise, athletes can suffer from glycogen deficiency, where most of the glycogen is spent from muscle. This can cause severe fatigue and difficulty moving. Glycogen deficiency can be alleviated by the constant consumption of high glycemic index carbohydrates, which will replace some of glucose during exercise. Special exercise regimens can also be used to make the muscle use a higher proportion of fatty acids for energy and thus less glycogen breakdown. Athletes can also use the method of carbohydrate loading, large amounts of carbohydrate consumption to increase glycogen storage capacity.
Examples of Glycogen Storage Diseases
There are two main categories of glycogen storage diseases: those caused by defective glycogen homeostasis in the liver and those caused by defective glycogen homeostasis in muscle. Diseases caused by impaired liver glycogen storage usually cause hepatomegaly (liver enlargement), hypoglycemia and cirrhosis (liver scarring). Diseases caused by impaired muscle glycogen storage often cause myopathies and metabolic disorders. Examples of glycogen storage diseases include Pompe Disease, McArdle Disease, and Andersen’s Disease.
Pompe Disease: Pompe disease is caused by mutations in the GAA gene that affect the skeletal and cardiac muscle, which encodes lysosomal acid alpha-glucosidase, also called acid maltase. Acid maltase is involved in the breakdown of glycogen, and disease-causing mutations result in harmful accumulation of glycogen in the cell. There are three types of Pompe disease: the adult, juvenile (juvenile) and increasingly severe infantile (childhood) form. The infantile form leads to death between the ages of one and two if left untreated.
McArdle’s Disease: McArdle disease is caused by mutations in the PYGM gene, which encodes myophosphorylase, the glycogen phosphorylase isoform found in muscle. Symptoms are mostly seen in children, but the disease may not be diagnosed until adulthood. Symptoms include muscle pain and fatigue, the disease can be life-threatening if not properly treated.
Andersen’s Disease: Andersen’s disease is caused by a mutation in the GBE1 gene, which encodes the glycogen branching enzyme, affecting muscle and liver. Symptoms usually appear around a few months of age and include growth retardation, liver enlargement, and cirrhosis. The complications of the disease can be life threatening.
In summary, glycogen, a highly branched polymer of glucose, is formed and broken down in most human tissues but is most abundant in the liver and muscle where it acts as a large stored fuel. Glycogen metabolism has been studied most thoroughly in liver and skeletal muscle. Glycogen metabolism in other tissues has not been studied so extensively, but is thought to resemble muscle process.